Common Supercapacitor Structure: Popular Models
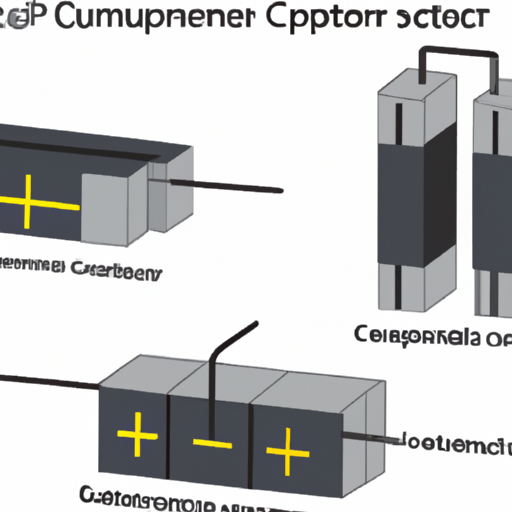
I. Introduction
Supercapacitors, also known as ultracapacitors or electrochemical capacitors, are energy storage devices that bridge the gap between traditional capacitors and batteries. They are characterized by their ability to store and release energy rapidly, making them essential components in various applications, from consumer electronics to renewable energy systems. This article aims to provide an in-depth understanding of supercapacitor technology, focusing on common structures and popular models, while also exploring emerging trends and future directions in the field.
II. Basics of Supercapacitor Technology
A. Explanation of Energy Storage Mechanisms
Supercapacitors store energy through two primary mechanisms: electrostatic double-layer capacitance and pseudocapacitance.
1. **Electrostatic Double-Layer Capacitance**: This mechanism occurs at the interface between the electrode and the electrolyte. When a voltage is applied, ions from the electrolyte accumulate at the electrode surface, forming an electric double layer. This process allows for the rapid storage of charge, resulting in high power density.
2. **Pseudocapacitance**: In addition to electrostatic storage, pseudocapacitance involves faradaic reactions, where charge is stored through the electrochemical oxidation and reduction of materials at the electrode surface. This mechanism enhances the overall capacitance and energy density of the supercapacitor.
B. Comparison with Traditional Capacitors and Batteries
Supercapacitors differ significantly from traditional capacitors and batteries. While capacitors store energy electrostatically and have low energy density, batteries store energy chemically and have higher energy density but slower charge/discharge rates. Supercapacitors combine the best of both worlds, offering high power density and rapid charge/discharge capabilities, making them ideal for applications requiring quick bursts of energy.
C. Key Advantages of Supercapacitors
1. **High Power Density**: Supercapacitors can deliver high bursts of power, making them suitable for applications like regenerative braking in electric vehicles.
2. **Long Cycle Life**: With the ability to withstand millions of charge/discharge cycles, supercapacitors have a significantly longer lifespan compared to batteries.
3. **Fast Charge/Discharge Rates**: Supercapacitors can be charged and discharged in seconds, allowing for quick energy delivery and replenishment.
III. Common Supercapacitor Structures
A. Electrolyte Types
The choice of electrolyte plays a crucial role in the performance of supercapacitors. Common types include:
1. **Aqueous Electrolytes**: These are water-based solutions that offer high ionic conductivity and are often used in EDLCs. However, they have a limited voltage window.
2. **Organic Electrolytes**: These electrolytes provide a wider voltage window and higher energy density but may have lower ionic conductivity compared to aqueous solutions.
3. **Ionic Liquids**: These are salts in liquid form that remain stable at high temperatures and voltages, offering excellent performance but at a higher cost.
B. Electrode Materials
The performance of supercapacitors is heavily influenced by the choice of electrode materials:
1. **Carbon-Based Materials**:
- **Activated Carbon**: Widely used due to its high surface area and low cost, activated carbon is the most common electrode material in supercapacitors.
- **Carbon Nanotubes**: These materials offer excellent electrical conductivity and mechanical strength, enhancing the performance of supercapacitors.
- **Graphene**: Known for its exceptional conductivity and surface area, graphene is a promising material for next-generation supercapacitors.
2. **Metal Oxides**: Materials like manganese oxide and ruthenium oxide can provide pseudocapacitance, increasing the overall energy density of supercapacitors.
3. **Conducting Polymers**: Polymers such as polyaniline and polypyrrole can also contribute to pseudocapacitance, offering flexibility in design and application.
C. Separator Materials
Separators are essential for preventing short circuits while allowing ionic transport:
1. **Porous Membranes**: These materials provide a physical barrier while allowing ions to pass through, ensuring safe operation.
2. **Non-Woven Fabrics**: Lightweight and flexible, these fabrics can be used as separators in various supercapacitor designs.
3. **Ion-Conducting Polymers**: These materials enhance ionic conductivity while providing structural integrity to the supercapacitor.
IV. Popular Supercapacitor Models
A. Electric Double-Layer Capacitors (EDLCs)
1. **Structure and Working Principle**: EDLCs utilize the electrostatic double-layer capacitance mechanism, with activated carbon electrodes and an electrolyte. When voltage is applied, ions accumulate at the electrode surface, creating a double layer that stores energy.
2. **Applications and Limitations**: EDLCs are widely used in applications requiring rapid charge/discharge cycles, such as in regenerative braking systems and power backup for electronic devices. However, they have lower energy density compared to pseudocapacitors.
B. Pseudocapacitors
1. **Structure and Working Principle**: Pseudocapacitors utilize faradaic reactions in addition to electrostatic storage. They often employ metal oxides or conducting polymers as electrode materials, allowing for higher energy density.
2. **Applications and Limitations**: Pseudocapacitors are suitable for applications requiring higher energy storage, such as in electric vehicles and renewable energy systems. However, they may have slower charge/discharge rates compared to EDLCs.
C. Hybrid Supercapacitors
1. **Structure and Working Principle**: Hybrid supercapacitors combine the features of EDLCs and pseudocapacitors, utilizing different materials for the positive and negative electrodes. This design allows for improved energy density and power density.
2. **Applications and Limitations**: Hybrid supercapacitors are versatile and can be used in various applications, including energy storage systems and electric vehicles. However, their complexity can lead to higher manufacturing costs.
V. Emerging Trends and Innovations
A. Nanostructured Materials
The development of nanostructured materials is revolutionizing supercapacitor technology. These materials offer increased surface area and enhanced electrochemical properties, leading to improved performance.
B. Flexible and Wearable Supercapacitors
As the demand for portable and wearable electronics grows, researchers are focusing on developing flexible supercapacitors that can be integrated into clothing and other materials, providing energy storage without compromising comfort.
C. Integration with Renewable Energy Sources
Supercapacitors are increasingly being integrated with renewable energy systems, such as solar and wind power, to provide efficient energy storage and management solutions.
D. Advances in Manufacturing Techniques
Innovations in manufacturing techniques, such as 3D printing and roll-to-roll processing, are enabling the production of supercapacitors with enhanced performance and lower costs.
VI. Applications of Supercapacitors
A. Automotive Industry
Supercapacitors are used in electric and hybrid vehicles for regenerative braking, providing quick bursts of energy to enhance performance and efficiency.
B. Consumer Electronics
In consumer electronics, supercapacitors are employed for energy storage in devices like smartphones and laptops, allowing for rapid charging and extended battery life.
C. Renewable Energy Systems
Supercapacitors play a crucial role in renewable energy systems, providing energy storage solutions that help balance supply and demand.
D. Industrial Applications
In industrial settings, supercapacitors are used for backup power, energy recovery, and in applications requiring high power output.
VII. Challenges and Future Directions
A. Limitations of Current Supercapacitor Technologies
Despite their advantages, supercapacitors face challenges such as lower energy density compared to batteries and higher costs associated with advanced materials.
B. Research and Development Trends
Ongoing research is focused on improving energy density, reducing costs, and developing new materials and structures to enhance performance.
C. Potential for Commercialization and Market Growth
As technology advances and costs decrease, the commercialization of supercapacitors is expected to grow, with increasing applications across various industries.
VIII. Conclusion
Supercapacitors represent a vital technology in the energy storage landscape, offering unique advantages that make them suitable for a wide range of applications. As research continues to advance, the future of supercapacitor technology looks promising, with potential for significant impact in energy storage solutions. Their ability to provide rapid energy delivery, long cycle life, and high power density positions them as a key player in the transition to sustainable energy systems.
IX. References
A comprehensive list of academic papers, industry reports, and relevant books and articles would be included here to support the information presented in the article.
---
This blog post provides a detailed exploration of supercapacitor structures and models, highlighting their significance in modern energy storage solutions.
Common Supercapacitor Structure: Popular Models
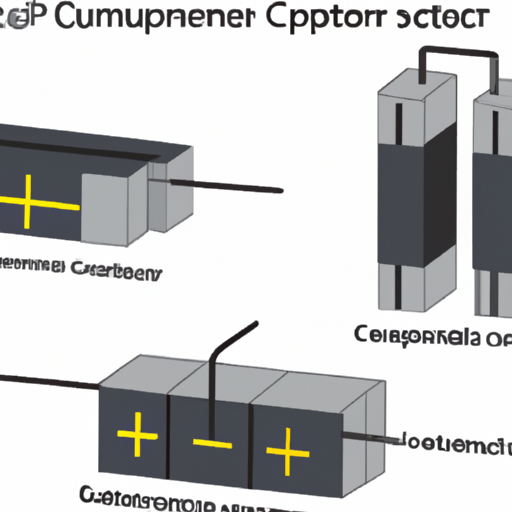
I. Introduction
Supercapacitors, also known as ultracapacitors or electrochemical capacitors, are energy storage devices that bridge the gap between traditional capacitors and batteries. They are characterized by their ability to store and release energy rapidly, making them essential components in various applications, from consumer electronics to renewable energy systems. This article aims to provide an in-depth understanding of supercapacitor technology, focusing on common structures and popular models, while also exploring emerging trends and future directions in the field.
II. Basics of Supercapacitor Technology
A. Explanation of Energy Storage Mechanisms
Supercapacitors store energy through two primary mechanisms: electrostatic double-layer capacitance and pseudocapacitance.
1. **Electrostatic Double-Layer Capacitance**: This mechanism occurs at the interface between the electrode and the electrolyte. When a voltage is applied, ions from the electrolyte accumulate at the electrode surface, forming an electric double layer. This process allows for the rapid storage of charge, resulting in high power density.
2. **Pseudocapacitance**: In addition to electrostatic storage, pseudocapacitance involves faradaic reactions, where charge is stored through the electrochemical oxidation and reduction of materials at the electrode surface. This mechanism enhances the overall capacitance and energy density of the supercapacitor.
B. Comparison with Traditional Capacitors and Batteries
Supercapacitors differ significantly from traditional capacitors and batteries. While capacitors store energy electrostatically and have low energy density, batteries store energy chemically and have higher energy density but slower charge/discharge rates. Supercapacitors combine the best of both worlds, offering high power density and rapid charge/discharge capabilities, making them ideal for applications requiring quick bursts of energy.
C. Key Advantages of Supercapacitors
1. **High Power Density**: Supercapacitors can deliver high bursts of power, making them suitable for applications like regenerative braking in electric vehicles.
2. **Long Cycle Life**: With the ability to withstand millions of charge/discharge cycles, supercapacitors have a significantly longer lifespan compared to batteries.
3. **Fast Charge/Discharge Rates**: Supercapacitors can be charged and discharged in seconds, allowing for quick energy delivery and replenishment.
III. Common Supercapacitor Structures
A. Electrolyte Types
The choice of electrolyte plays a crucial role in the performance of supercapacitors. Common types include:
1. **Aqueous Electrolytes**: These are water-based solutions that offer high ionic conductivity and are often used in EDLCs. However, they have a limited voltage window.
2. **Organic Electrolytes**: These electrolytes provide a wider voltage window and higher energy density but may have lower ionic conductivity compared to aqueous solutions.
3. **Ionic Liquids**: These are salts in liquid form that remain stable at high temperatures and voltages, offering excellent performance but at a higher cost.
B. Electrode Materials
The performance of supercapacitors is heavily influenced by the choice of electrode materials:
1. **Carbon-Based Materials**:
- **Activated Carbon**: Widely used due to its high surface area and low cost, activated carbon is the most common electrode material in supercapacitors.
- **Carbon Nanotubes**: These materials offer excellent electrical conductivity and mechanical strength, enhancing the performance of supercapacitors.
- **Graphene**: Known for its exceptional conductivity and surface area, graphene is a promising material for next-generation supercapacitors.
2. **Metal Oxides**: Materials like manganese oxide and ruthenium oxide can provide pseudocapacitance, increasing the overall energy density of supercapacitors.
3. **Conducting Polymers**: Polymers such as polyaniline and polypyrrole can also contribute to pseudocapacitance, offering flexibility in design and application.
C. Separator Materials
Separators are essential for preventing short circuits while allowing ionic transport:
1. **Porous Membranes**: These materials provide a physical barrier while allowing ions to pass through, ensuring safe operation.
2. **Non-Woven Fabrics**: Lightweight and flexible, these fabrics can be used as separators in various supercapacitor designs.
3. **Ion-Conducting Polymers**: These materials enhance ionic conductivity while providing structural integrity to the supercapacitor.
IV. Popular Supercapacitor Models
A. Electric Double-Layer Capacitors (EDLCs)
1. **Structure and Working Principle**: EDLCs utilize the electrostatic double-layer capacitance mechanism, with activated carbon electrodes and an electrolyte. When voltage is applied, ions accumulate at the electrode surface, creating a double layer that stores energy.
2. **Applications and Limitations**: EDLCs are widely used in applications requiring rapid charge/discharge cycles, such as in regenerative braking systems and power backup for electronic devices. However, they have lower energy density compared to pseudocapacitors.
B. Pseudocapacitors
1. **Structure and Working Principle**: Pseudocapacitors utilize faradaic reactions in addition to electrostatic storage. They often employ metal oxides or conducting polymers as electrode materials, allowing for higher energy density.
2. **Applications and Limitations**: Pseudocapacitors are suitable for applications requiring higher energy storage, such as in electric vehicles and renewable energy systems. However, they may have slower charge/discharge rates compared to EDLCs.
C. Hybrid Supercapacitors
1. **Structure and Working Principle**: Hybrid supercapacitors combine the features of EDLCs and pseudocapacitors, utilizing different materials for the positive and negative electrodes. This design allows for improved energy density and power density.
2. **Applications and Limitations**: Hybrid supercapacitors are versatile and can be used in various applications, including energy storage systems and electric vehicles. However, their complexity can lead to higher manufacturing costs.
V. Emerging Trends and Innovations
A. Nanostructured Materials
The development of nanostructured materials is revolutionizing supercapacitor technology. These materials offer increased surface area and enhanced electrochemical properties, leading to improved performance.
B. Flexible and Wearable Supercapacitors
As the demand for portable and wearable electronics grows, researchers are focusing on developing flexible supercapacitors that can be integrated into clothing and other materials, providing energy storage without compromising comfort.
C. Integration with Renewable Energy Sources
Supercapacitors are increasingly being integrated with renewable energy systems, such as solar and wind power, to provide efficient energy storage and management solutions.
D. Advances in Manufacturing Techniques
Innovations in manufacturing techniques, such as 3D printing and roll-to-roll processing, are enabling the production of supercapacitors with enhanced performance and lower costs.
VI. Applications of Supercapacitors
A. Automotive Industry
Supercapacitors are used in electric and hybrid vehicles for regenerative braking, providing quick bursts of energy to enhance performance and efficiency.
B. Consumer Electronics
In consumer electronics, supercapacitors are employed for energy storage in devices like smartphones and laptops, allowing for rapid charging and extended battery life.
C. Renewable Energy Systems
Supercapacitors play a crucial role in renewable energy systems, providing energy storage solutions that help balance supply and demand.
D. Industrial Applications
In industrial settings, supercapacitors are used for backup power, energy recovery, and in applications requiring high power output.
VII. Challenges and Future Directions
A. Limitations of Current Supercapacitor Technologies
Despite their advantages, supercapacitors face challenges such as lower energy density compared to batteries and higher costs associated with advanced materials.
B. Research and Development Trends
Ongoing research is focused on improving energy density, reducing costs, and developing new materials and structures to enhance performance.
C. Potential for Commercialization and Market Growth
As technology advances and costs decrease, the commercialization of supercapacitors is expected to grow, with increasing applications across various industries.
VIII. Conclusion
Supercapacitors represent a vital technology in the energy storage landscape, offering unique advantages that make them suitable for a wide range of applications. As research continues to advance, the future of supercapacitor technology looks promising, with potential for significant impact in energy storage solutions. Their ability to provide rapid energy delivery, long cycle life, and high power density positions them as a key player in the transition to sustainable energy systems.
IX. References
A comprehensive list of academic papers, industry reports, and relevant books and articles would be included here to support the information presented in the article.
---
This blog post provides a detailed exploration of supercapacitor structures and models, highlighting their significance in modern energy storage solutions.